Meet a difference maker…in clean water – Professor Philip Davies
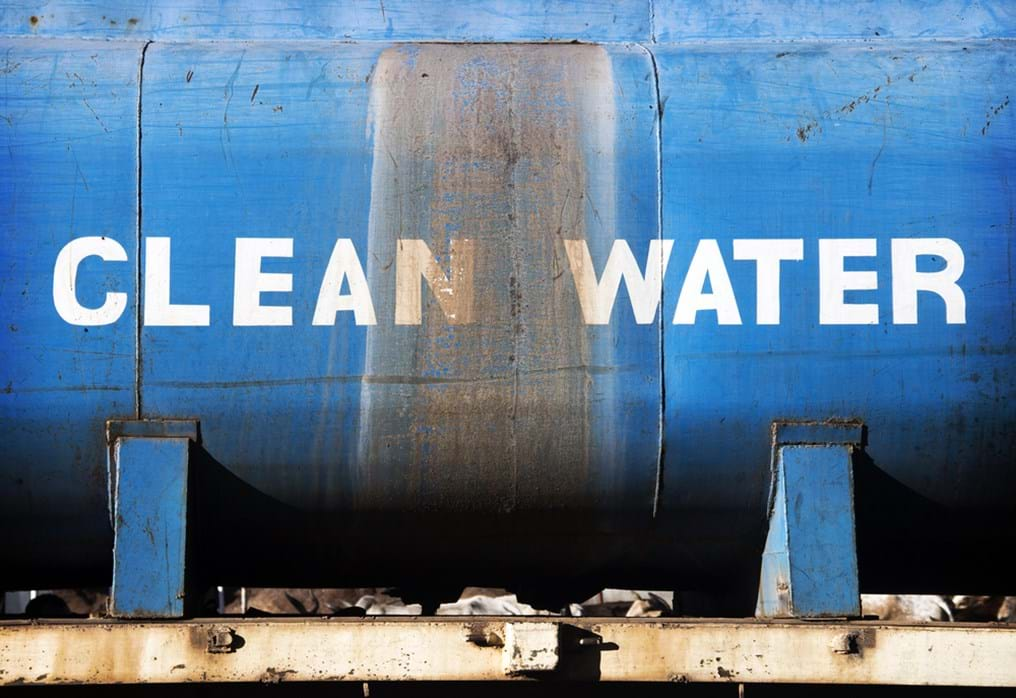
22nd March 2022
In this new blog series, we’re speaking to chemical engineers across the world making a difference to make sustainable practices and products a reality and more accessible to all for the wider benefit of our society and globe.
This is part of our recently launched Sustainability Hub, which is a giving chemical engineers access to a growing suite of new on-demand training courses and knowledge resources to help them to embed sustainable principles and practices into everyday work and life.
Today on World Water Day (22 March 2022), Professor Philip Davies shares his work in water desalination, and particularly his unique process combining reverse osmosis with renewable energy sources to create safe drinking water from seawater in arid countries.
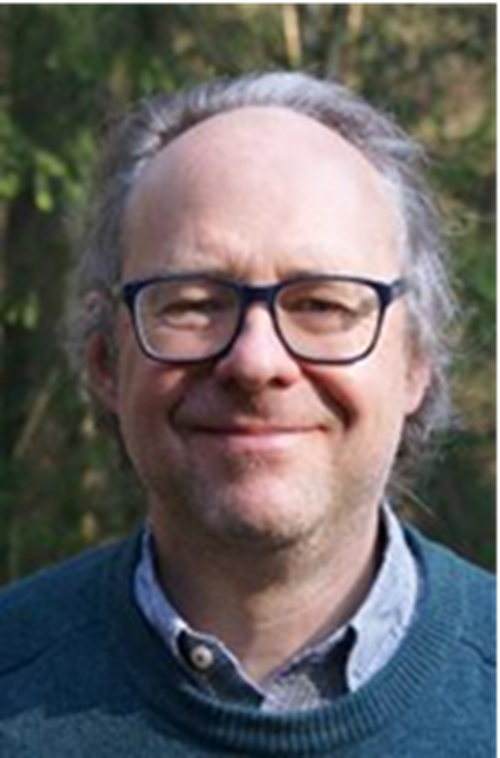
Name: Professor Philip Davies
Job title and organisation: Professor of Water Technology, the University of Birmingham, UK
Bio: Philip has pursued a varied industrial and academic research career, including projects in solar energy, desalination, solar-powered cooling, and seawater greenhouses. His current research at the University of Birmingham, UK focuses on technologies to help with issues of resource scarcity in arid regions.
Tell us about your career in desalination, water and wastewater treatment, and how your research into reverse osmosis with renewable energy systems came about?
I first became interested in water desalination when working on an EU-funded project for a company called Seawater Greenhouse, which had a pilot installation in Tenerife, Spain in 1993. This project was about using renewable energy together with desalination and built on interests I had developed in solar energy conversion while working on an earlier EU project based at the Polytechnic University of Madrid in Spain.
Since then, I have worked on diverse technologies and application fields, but what fascinated me about these early projects was the absolute constraint that water places on life in arid environments – especially on an arid island with limited options for sourcing or transporting water from other geographical locations.
Islands like Tenerife were once agricultural paradises, but that’s no longer the case, and they can be seen as a microcosm of desertification and water scarcity seen now at much wider scale. Together with the availability of sunlight, I feel there is an important opportunity to desalinate seawater of which there is no shortage.
A second direction was about engagement with Indian researchers and becoming interested in the water issues of the Indian subcontinent. I got invited to a seminar at the Indian Institute of Technology (IIT) in Delhi where affordable water treatment for rural areas was discussed.
About that time, I also became aware of the possibilities of natural treatment systems for wastewater, which are especially interesting for rural economies where land is relatively abundant and there is little chance to connect to centralised sewerage systems and treatment plants.
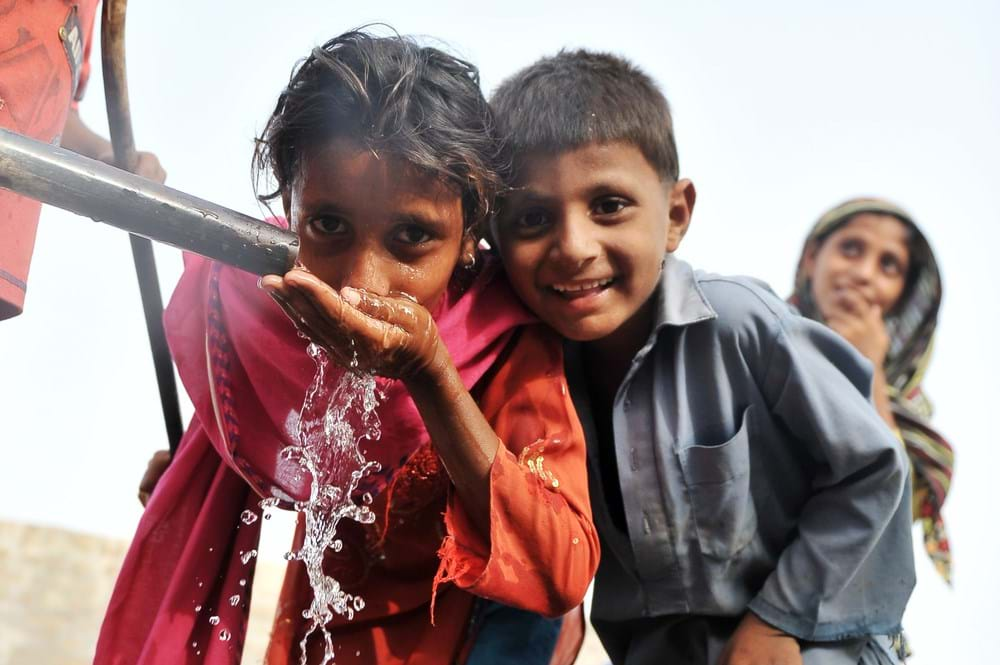
A further interest that developed from working with my new connections in India, was that of membrane-based desalination systems - which is the process by which salt and minerals are removed from water solution when it passes through a semipermeable membrane.
We got some joint UK-India funding that enabled us to set up a laboratory reverse osmosis system for use with renewable energy sources. Reverse osmosis is a water purification process that uses a partially permeable membrane to separate ions, unwanted molecules and larger particles from drinking water. So by researching and testing this with renewable energy systems, using sources of solar and biomass energy, we were able turn the seawater into safe drinking water, using energy systems that are reliable, cost-effective, efficient and sustainable.
This technology is very much needed in India where groundwater is heavily relied on but frequently is contaminated by salts and other pollutants above save limits. Reverse osmosis is a good solution to remove salts as well as more modern pollutants such as pharmaceutical residues, antibiotics and pesticides which are toxic even at very low levels.
It is always exciting to know how engineers are pushing the boundaries to achieve a net-zero future. Could you explain how your innovative reverse osmosis process reduces energy consumption and carbon?
Currently, desalination is used mainly in wealthy countries to supply potable water. Reducing the energy consumption of the process is essential to enable future scale-up and meet broader water needs, such as agriculture.
Reverse osmosis is the most efficient desalination process, using at least ten times less energy than older technologies based on distillation, but its energy footprint is still on the high side – if it were to be scaled up massively the carbon footprint would be unacceptable. As a result, my team’s research on reverse osmosis has focused on making this process more efficient.
Our work on this topic went through several iterations. The early iterations were more focused on the energy source, especially low-grade heat sources, like solar and biomass energy, and how to couple them to the reverse osmosis process.
However, we also realised that it was possible to improve the configuration of the process itself, to reduce its energy consumption in ‘high-recovery’ applications. These are applications where it’s essential to recover as much clean water from the feedwater as possible – which is typically important in groundwater and water reuse.
This led on to the batch reverse osmosis system, which is the system we’re developing today at the University of Birmingham. Using our system, we can recover as much as 95% of water with an energy consumption of less than 1 kWh of electricity per cubic metre of water produced. This contrasts with most standard systems, which only recovery about 60-70% of water, meaning that the remaining 30-40% is wasted as discarded as brine. This means wastage of the source water.
In the case of groundwater, such wastage results in more rapid draw down of wells and further depletion of aquifers. This is a big problem in the Punjab where groundwater has fallen drastically in recent years, and has to be pumped from huge depths to maintain irrigation of staple crops like rice and wheat. Another example of groundwater depletion is occurring along the coastal strip of Gujarat, where industrial and farming activities have gradually caused water levels to drop and seawater to infiltrate. The resulting salinisation of aquifers is making farming less and less viable because of low yields and poor quality of crops.
High recovery desalination is also important in industrial applications where the objective is not only to recover water but also the mineral component of the feedwater. Examples here include the electroplating industry where valuable metals get diluted in successive operations and finally have to be disposed of at great cost. That can be avoided by reconcentration using reverse osmosis equipment. A recovery of 95%, for example, means that the minerals get concentrated 20 times into just 5% the initial volume, which is very important when it comes to recovering and reusing them. This helps to make industrial users less vulnerable to hikes in prices of special metals, such as chromium, nickel, and lithium, which are sourced through complex international supply chains subject to geopolitical disruption though wars, such as the war in the Ukraine. These materials underpin applications such as energy storage, electronics for power conversion and high-efficiency electric motors.
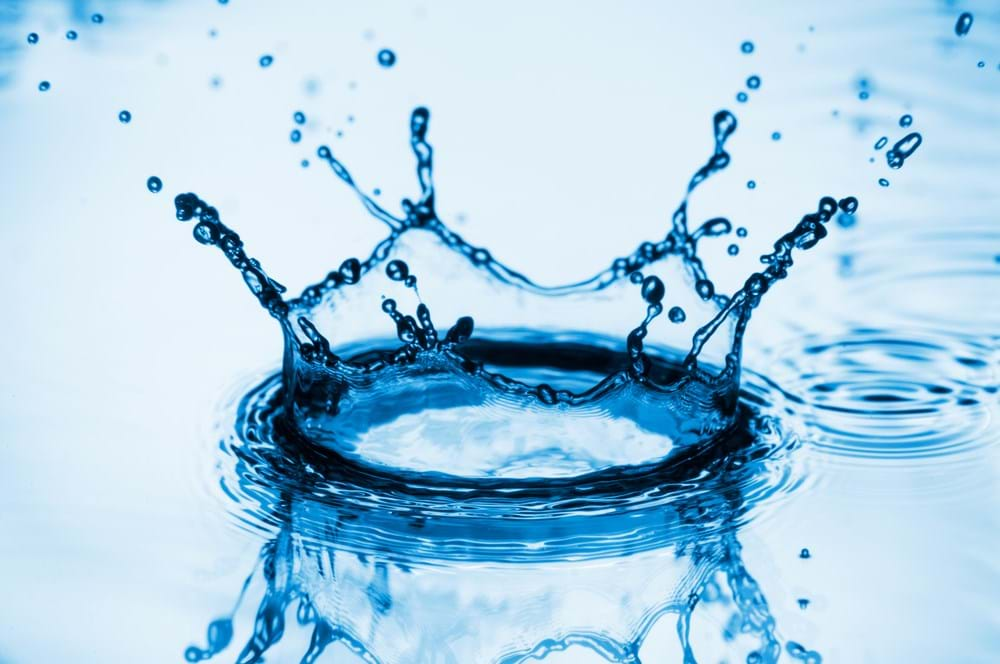
As we as a profession are aiming to achieve an inclusive, diverse, and equal society, part of the job for engineers is to promote and raise the standard of sustainable practices for developing countries. Can you tell us about your H2O project in India where your team championed to prevent water scarcity by creating innovative processes to provide fresh, reliable drinking water?
Yes indeed, sadly global challenges such as water scarcity and climate change impact most significantly on those least responsible and least resourced to deal with the consequences. This includes minority groups such as women in developing countries.
Of course, though considered a lower/middle income country (LMIC), India also has many advantages like a rapidly growing low-cost manufacturing base, and many well-educated young engineers and scientists.
In the water sector, they are particularly well-placed to develop low-cost sensors and affordable smart monitoring systems. In the India H2O project, there are 18 Indian and European partners working together to provide low-cost innovative water treatment processes.
Eventually, these should have benefits to both developing and developed countries alike.
The India H2O project covers a range of innovations in advanced membrane processes and in natural treatment systems. The advanced membrane processes include reverse osmosis for groundwater desalination and forward osmosis for water reuse from municipal and industrial effluents.
The natural treatment systems make use of both freshwater- and salt-tolerant plants such as the Salicornia species, which grow in salt marshes, beaches and mangroves. Not only can such plants manage difficult waste streams like brines from desalination plants, but they can also provide a nutritional crop.
So alongside drinking water production, there will be many benefits in terms of reuse of resources and avoiding dumping polluting effluents into the environment.
How do you think your chemical engineering skills and expertise contributed to the success of this project?
Water technology is an interdisciplinary area requiring skills from areas including chemical engineering, civil engineering and environmental engineering.
In the design of our desalination systems, the well-known approaches of chemical engineers – including mass and energy balance, mass transfer, and chemical thermodynamics - were used. Thermodynamics provides a benchmark to the performance of the process, in the sense that we constantly compare the energy consumption to the ideal minimum to see how far off we are and what improvements may be possible.
As the equipment gets scaled up and pressure requirements have increased, safety and hazards become a matter of increasing concern.
So we’ve used systematic approaches of chemical engineer to assess and manage such risks.
Visit our Sustainability Hub to learn about others making a difference in our Sustainability success stories.